Lee Creates New Microfluidic Platform for Intracellular Delivery
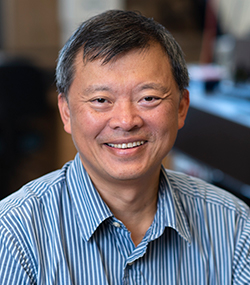
Dec. 8, 2021 – Cell therapy has become a powerful tool to treat patients suffering from cancer and other debilitating diseases stemming from gene mutations. One of the critical steps to manufacturing cell therapy products is the intracellular delivery of genetic coding molecules to make cells more powerful in fighting diseases.
To address the challenges of intracellular delivery, UCI researchers led by Abraham Lee have developed a microfluidic platform to safely and precisely deliver genetic coding molecules into cells. Called Acoustic-Electric Shear Orbiting Poration (AESOP), their high-throughput nonviral intracellular delivery platform optimizes transfer of cargo sizes with poration, or pore formation, of the cell membranes.
Lee, senior author, Samueli School professor of biomedical engineering and director of the Center for Advanced Design and Manufacturing of Integrated Microfluidics; and co-first authors Mohammad Aghaamoo, recent Ph.D. graduate and current postdoctoral researcher in biomedical engineering; and Joy (Yu-Hsi) Chen, doctoral candidate in biomedical engineering, have published their research in the Oct. 29, 2021, issue of Advanced Science.
The standard approach for intracellular delivery is to use the molecular machinery of viruses to deliver genetic molecules into cells. However, viral delivery is limited by the size of the molecules it can carry. It also suffers from unwanted side effects from the virus that can be harmful to the patient.
“The ability to achieve intracellular delivery without the use of viruses is a holy grail for many applications, including but not limited to cell therapy – drug discovery processes, stem cell research, gene editing, etc.,” said Lee. “Although there are other nonviral methods, ours is unique in the sense that it can deliver molecules precisely and uniformly to a large population of cells, enabling safe and more effective cell engineering for therapeutic purposes.
“Our technology relies on a series of vortices, which are analogous to micro ‘washing machines,’ that stretch and twist the clothes (i.e., the cells) to make sure every part of it is uniformly absorbing the detergent (i.e., the genetic coding molecules).”
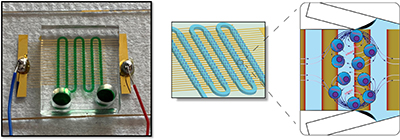
The vortices are formed based on tiny air bubbles trapped in lateral side cavities, something Lee’s lab has developed called lateral cavity acoustic transducers or LCATs. By applying an acoustic energy to the device, the microvortices are formed. Researchers also fabricate electrodes beneath the vortices to apply electrical fields. Lee’s group has demonstrated uniform and precise cell transfection – a procedure introducing foreign nucleic acids into cells to produce genetically modified cells, shown by the intracellular delivery of DNA plasmid, small double-stranded molecules, as well as the gene editing of cells with large genetic-coding plasmid complexes.
“Our microfluidic platform, AESOP, can uniformly create pores of given sizes on the membranes of a large number of cells simultaneously,” said Lee. This uniform pore formation allows a gentler disruption of all cell types, since pore sizes can be tailored to the right size for different types of molecules. This uniform mixing between the cells and the molecules induced by the vortices dictates that the minimum number of molecules are delivered, further enhancing the survivability of the cells during the process. As a result of the combination of these technologies, the cells are uniformly and precisely engineered for a wide variety of therapeutic applications.
Adoptive cell therapy (ACT) is one of the most promising types of cell therapy, Lee explained. ACT involves drawing blood from patients, separating targeted immune cells, delivering genetic material into the cells, then expanding the cell population before re-introducing them into the patient to boost their immunity specifically tailored for the disease.
“Near ‘cures’ based on ACT have been found for several blood cancers,” said Lee. “By improving the intracellular delivery to make it safer and more precise, it will enable a wide range of applications and hopefully transform many other medical therapies that rely on safe delivery of genetic coding molecules into cells.”
Other contributors include UCI biomedical engineering researchers Xuan Li, Neha Garg, Ruoyu Jiang, and Jeremy Tian-Hao Yun.
Research was funded by the National Science Foundation and the Center for Advanced Design and Manufacturing of Integrated Microfluidics.
– Tonya Becerra